New technologies are rapidly being connected to the electricity system. The connection of these technologies (think wind, solar, storage, etc.) needs to be planned to make the whole electricity system work. The planning of electricity systems has been a relatively traditional process of studies and modeling. However, in recent years, a change has occurred, a recognition that zero emission resources are cheaper than thermal-fossil generation. This fact unlocks some advantages and challenges never faced by the electricity planning process before. The biggest advantage is that there is a pathway available to reduce emissions in the electricity sector, while reducing the cost.
The knowledge that reducing emissions and cost is simultaneously available for the electricity sector coincides with the recognition that anthropogenic climate change is driven primarily by burning fossil fuels in the production of energy. The problem is, however, that electricity is not the entire source of greenhouse gas (GHG) emissions, rather it is only a portion. In the figure below, I show the share of U.S. emissions among sectors for 2017.
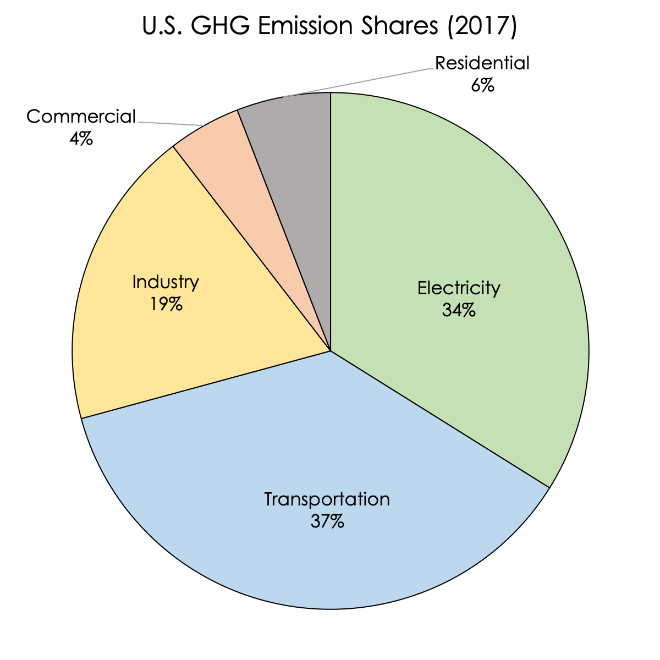
Figure 1: The GHG emission shares for the United States in 2017. Total emissions were 5,143.8 million metric tons. Image data source EIA.
Since electricity produces only approximately a third of the total emissions, the other sectors also need to reduce their emissions to help mitigate climate change. The issue is that the electricity sector is the only sector that has a viable pathway today for reducing emissions at low-cost (compared to alternatives). Thus, the answer becomes electrification. This refers to the process of removing an asset that is consuming a fossil fuel for its energy needs and replacing it (or repurposing it) to use electricity for its energy needs. The example that most people are familiar with is buying an electric vehicle to replace an internal combustion engine vehicle.
During the process of electrification, new strains and opportunities are transferred to the electricity sector. The rate of transition to electrify other sectors, and the amount which can be electrified, all need to be considered. This results in the paradigm of “Planning for Integrated Energy Systems”.
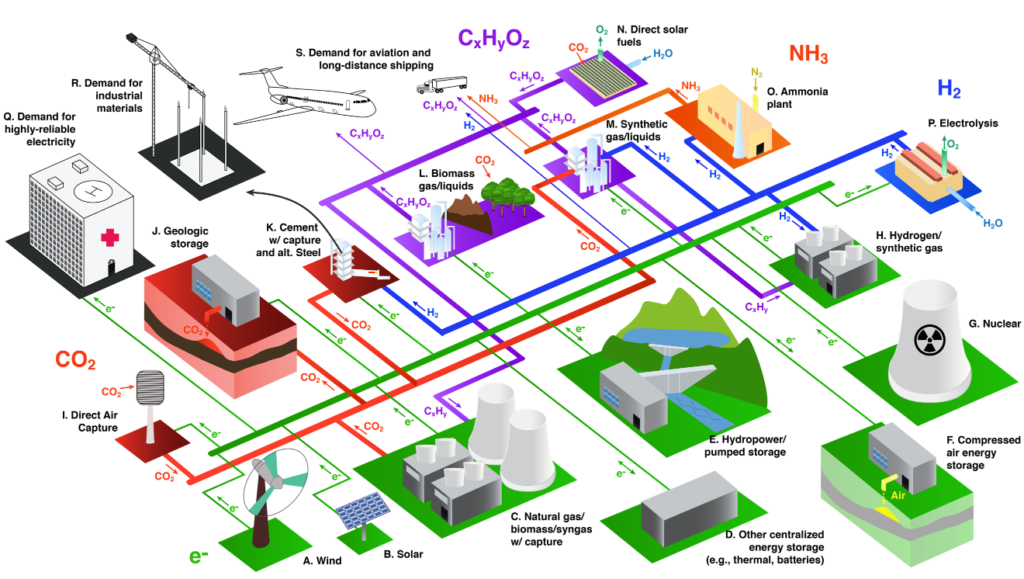
Figure 2: A conceptual image of an integrated energy system that can decarbonize the economy. Image from “Net-zero emissions energy systems”, Davis et al., Science, 2018 (https://doi.org/10.1126/science.aas9793).
Figure 2 illustrates a hypothetical energy system that could provide energy services while emitting zero GHGs. The image is from Davis et al., 2018 (I was a co-author). The traditional electricity system is denoted in green. These electricity resources would be connected together by transmission, which can be co-optimized to improve the benefits of resource sharing, geographic diversity and demand smoothing. The wind and solar power plants are driven by the weather and it is critical to model these with high resolution data over large scales (both space and time). Further, the weather data should be included to parameterize the transmission path rating, heat rates of thermal power plants, and changes in demand.
The services that are being provided by electricity could include EVs, commercial and residential heating for space and water, industrial loads, and hydrogen production (for medium- and heavy-duty transportation). To plan for these new electricity demands requires modeling of their behavior with high-fidelity. For EVs, some immediate questions arise: When do people charge their vehicles? Where do the chargers need to be sited? What rating should they be? How much additional demand do the EVs add to the traditional demand? The same process needs to be performed for water heating, space heating, and the industrial loads. These new demands are difficult to parameterize perfectly because the demands currently exist at very low levels. Trying to predict how these loads will manifest in the electricity system is an emerging field of research.
Hydrogen production (as well as ammonia and synthetic fuel production) must be modeled with the parameters for building the facilities to produce the fuel, the transport infrastructure to move the fuel, and storage facilities to keep the fuel locally. The fuel production will need to have electricity supplied – this provides one of the most flexible resources on the demand side.
With the electrification process described above, the new demands come with one of the final critical features of planning for integrated energy systems: flexibility. The demand-side can be dispatched within the energy system, so that instead of simply supplying generation for a fixed demand, the integrated system can reduce energy consumption to balance itself. These demand-side resources can be compensated for their participation. Part of the demand-side flexibility could also come from behind-the-meter solar and storage.
Therefore, to plan an integrated energy system, modeling needs to reflect all the different resources described above – demand-side, behind-the-meter, variable resources, transmission, grid-scale storage, and new demand profiles (spatially and temporally). These all need to include high-resolution weather data because it will be a main driver of demand and supply. Further, the modeling platform needs to be flexible enough to be expanded for new technologies, policies, and social concerns.
These types of models for planning integrated energy systems are in short supply because they are complex, computationally expensive, and require multiple disciplines in their development. However, they are incredibly important because they enable a glimpse of possible futures that may exhibit very different properties from the past. The emergent properties could be counter-intuitive, guide new policies, or highlight possible issues or challenges that lower-fidelity models might not identify. These new models will be indispensable in helping to understand the impacts and guide the direction of new energy infrastructure investments as we move towards a low-carbon future.
Dr. Christopher Clack
Vibrant Clean Energy, LLC
This is clearly a simplified model, which assumes correctly the extent of the existing electric network[s]. However, networks for both hydrogen [gas] and CO2 [gas] are effectively non-existent, where production & portability of such within a liquid form [to overcome lack of network limitations] follows steep energy intensitivities CO2<<<CH4<<<<<H2, owing largely to temperature of liquid form. When it comes to scaled production [liquefaction], infrastructure deployment & “non-pipeline alternatives”, we see LNG as a lessons learned model for both CO2 [albeit EOR has 40 years demonstrating network/market] & H2. Does the above model, seek largely to centralize both CO2 (via CC-US) and H2 [via electrolysis] production, say within “industrial zones”, for utilization within same geographic/industrial zones, to limit a) high CapEx of pipeline networks &/or b) high CapEx & EnergyEx for liquefaction/portability of CO2 &/or H2?
We’ve already seen many “pilots” on the CO2 – CC-US/EOR front, with relatively dismal success & longevity. We are now seeing constant bombardment of a Hydrogen Economy “Renaissance” – predicated on “excess, variable RE” electrolysis; usurping the NG midstream & downstream/distribution networks to make it “distributed”; fuelcells – just don’t ask were the H2 comes from [methane reformation]; and my favorite – a globally portable LH2 supply chain [i.e. Australia [or BC] to Japan & S Korea], ignoring all CapEx/EnergyEx constraints whatsoever.
I can envision this happening within a centralized structure, powered via “RE” [low carbon] electrons arriving by the electric network, but not if we attempt/pursue turning H2 into a globally mobile energy carrier commodity with “distributed” penetration throughout society.
I would like to add one additional method of storage: heat storage tank )molten salt) off of nuclear reactor.